Why
aren't my
stars round?
How to figure out what is wrong with your telescope when
stars
appear distorted (updated
Aug. 2016)
All images and text Copyright Mike
Lockwood, 2016
So
you've had a telescope for a while, or just gotten a new one, and you
notice consistent or inconsistent distortion in the star images.
To put it another way, stars aren't round in the
eyepiece
inside or outside of focus, or maybe even at best focus. This
may be intermittent, or may always be the case.
How do you figure out the source of the distortion? Is the
problem mechanical, or are the optics flawed?
I hope
this article is helpful in leading you down a path that will lead to an
explanation.
There are many possible causes of distorted, non-circular star shapes.
You
may even be seeing more than one
distortion on top of
another one or two! This can make deciphering
the puzzle a
bit
difficult, but hopefully this article will allow you to identify one
problem
fairly easily, and then after fixing it you will be able to track down
the other.
In order for this article to make sense, first we have to set some
ground rules and satisfy a few conditions before we can move into
diagnosis of the problem(s).
First
condition:
For all of the
advice to follow, please put the star in the center of the field of
view and keep it there.
This is important for determining if the issue is due to
collimation, and reduces the chance of some other eyepiece or corrector
aberration being confused with another issue.
Second
condition:
Before you do anything else, determine if you have
astigmatism or other distortion in your eyes.
This is fairly simple - if the stars in the telescope do not
appear to be round, tilt/turn
your head. Distortion in star images that is caused by distortion
in
your eyes will rotate with your head. You may even
already know from your eye doctor that you have
astigmatism. However, if you don't rule out this
problem first, you may drive yourself crazy
trying to figure out what is going on!
Third
condition:
Next, take a look at what
power you are using - if it is fairly low, the distortion may be coming
from the pupil of your own eye vignetting the light cone. Move
your eye side to side while looking at a focused and defocused star -
if the
shape
changes or the secondary shadow appears to move around, your own pupil
is blocking some of the light and may be
causing the strange shape you are seeing. In this case,
either 1)
keep your eye carefully centered over the eyepiece, 2) raise
the
power, and/or 3) get more dark adapted so your pupil is larger
and
does not
block the light cone. If you are already using high power,
you
may
wish to try low power to see this effect for yourself and know what it
looks like. Your friends will thank you when you point this
out
to them.
Fourth
condition: If it's
early in the evening, understand that your mirror will
likely be cooling off, and strange things can happen.
Air
currents can form, cooling parts of the mirror faster than others.
A breeze can set up asymmetric airflow in the telescope's
tube or
mirror box, also cooling some of the mirror faster than the rest.
Both of these are a recipe for strange star shapes that go
away
after a bit of cooling. This goes for the primary mirror
and/or
the secondary, especially if it's fairly large.
Fifth condition:
For this article,
we are assuming that your mirror cell is properly designed and set up,
including the edge support of the mirror. If
your optics are
glued to the cell, then you cannot easily rotate them to help rule out
some possible causes of non-round stars. Gluing a mirror
(other
than a small secondary) to a
cell made of something other than glass will often cause it to warp as
the cell shrinks by a different amount and pulls on the mirror,
creating optical problems. I do not recommend
gluing large
mirrors to their cells/holders, as it will often cause non-round stars.
If your primary mirror and or large secondary mirror is glued
to
something, I recommend finding a way to mount it without glue, and then
coming back to this article if problems remain.
Now, understanding the possibilities given above, let's begin.
Collimation and
coma in a Newtonian telescope
By
far the most
common reason for non-round stars in a Newtonian telescope is poor
collimation. This produces an optical aberration called
coma.
Some examples of the appearance of coma in images can
be
found in the diagram to the right. Keep in mind that coma may
be
combined with other optical problems.
With
severe coma, stars appear distinctly comet-shaped. With less
severe coma, stars still appear asymmetric at or near focus, a
characteristic that we will use to our advantage.
If you don't know what coma
looks like, remove
the coma corrector from your telescope if you are using one, put in a
high-quality eyepiece, move the star
around the field, and see coma for yourself.
With
no
coma corrector in place, a star placed precisely in the center
of the
eyepiece's field
of view shouldappear
round if the telescope is collimated properly. If it is not,
there will be a spot somewhere else in the field where the star appears
round. This is because a Newtonian telescope will produce
a
perfectly
round star image at the center of the field if it is collimated
properly.
However, if the telescope (without coma
corrector) is moved so
that the
star is moved to the
right of the center of the field of view, you will see the star start
to bulge, or it may appear to grow a tail that points toward the right.
Likewise, on the left of the center of the field of view,
the
bulge or
tail
will point to the left. In fact, no matter where the star is,
the
bulge/tail will always
point
away from the center of the field, except at the exact center of the
field,
where the tail does not exist. Move the star around the field and
observe this effect. You will see more coma the faster the
telescope is.
Defocus the star in both directions - inside and outside of
focus. Coma will remain on the same side of the star and will not
reverse on the other side of focus. Other distortions such as
astigmatism or pinched optics will appear to rotate or flip on the
other side of focus.
Now that you know what coma looks like, I will describe how I collimate
a telescope. First, though, here are some points that I need to
make.
1) NOTE:
IF YOU SPEND AN HOUR OR TWO LEARNING THE COLLIMATION
TECHNIQUE
DESCRIBED BELOW, YOU MAY IMPROVE YOUR TELESCOPE'S IMAGES
GREATLY
WITHOUT HAVING TO SPEND A CENT ON NEW COLLIMATION TOOLS.
You will
also have a sharper eye for collimation than most other observers.
2) NOTE: Lasers can
easily go out of alignment, and this will
seriously throw off telescope collimation.
Put
your laser in the
focuser and push the top of the laser against the top of the focuser.
I don't usually tighten the thumbscrews, because they often
tilt the laser within the focuser. Now rotate the laser in
the focuser (you can also do this in a v-block). If the laser
spot moves in a circular
path on the mirror, then the laser needs to be aligned.
Consult a knowledgeable
astronomer or the manufacturer to get help with this. Also,
rack
the focuser in and out - the laser spot should not move. If
it
does, the focuser has issues.
Relating to the
focuser, here are some other mechanical tips:
Do all of your collimating with the focuser racked all the way in and
then backed off the slightest bit so that the stops are not putting
pressure on the drawtube.
Make sure the focuser base is snug on the focuser board. Next
check the focuser attachment to the base plate - if the focuser is not
seated firmly on the base it may shift a bit. You can loosen the
two setscrews that hold the focuser in the base and remove it.
Clean the base of the focuser and top and inside of the base plate so
make sure that no grit is in there. Put the focuser back in the
plate and press it firmly against the plate while re-tightening the two
setscrews. Additionally, for the SIPS, when doing initial
positioning tighten the focuser and locking ring as a unit - you will
get a better connection.
Clean the top of the focuser drawtube and the underside of the lip of
the laser collimator. These two surfaces meet when the laser is
inserted. Push the laser against the focuser - that makes it
contact against two machined surfaces and should align the laser and
focuser axes. You can spin the laser in the focuser, maintaining
a little pressure to keep the laser against the top of the
focuser. As I said above, the dot should not move when the laser
is rotated, though it may oscillate a little bit.
When you gently tighten one thumbscrew, make sure the laser dot doesn't
move. If it does, the thumbscrew is tilting the laser in the
focuser tube, and this will throw off your collimation. Sometimes
I don't tighten the thumbscrew at all. Sometimes I gently snug up
one or the other or both. It depends on the fit of the laser in
the focuser, and this can be different for every laser and
focuser! Get to know yours, and know what you have to do to have
the laser not moving around in the focuser tube while also not having
the collimation thrown off.
3) NOTE: Using
only collimation tools - laser, barlowed laser,
cheshire, autocollimator, etc., I almost never achieve perfect
collimation. That is, I can almost always detect
some coma in a
star image using the technique described below. So, I always
finish up collimation using a star image, and that is what I will
describe here.
With those points in mind, we can now start collimating.
Go through your normal
collimation procedure, without a coma
corrector,
paying particular
attention to making the secondary mirror alignment as close to perfect
as you can get it.
Let's call this "rough collimation", and many people don't do anything
further after it, but we will. You will probably use a laser for
this process. If you need help with this, seek help elsewhere or
from a
friend.
If you can nail the secondary alignment, the instructions
below will then help you get close to perfect primary and system
collimation.
From
here on, we begin "fine collimation", done using a star image, a
technique that I have used for many years.
Put your coma corrector back in the focuser
if the telescope is f/5 or below. Put in a fairly
high-power eyepiece, put a star in the center of the field of
view, and keep
it
there using a drive or by hand-tracking.
But wait, why are we using the coma corrector? Aren't we supposed
to collimate without it? The answer is yes, but only when using a
laser. We SHOULD use the coma corrector it for "fine
collimation", which done using a star image.
Using the coma corrector will correct, or cancel, nearly all of the
coma that the telescope would normally have without it. However, if the primary mirror
is not collimated properly, residual coma due to
mis-collimation will still be seen even with the coma corrector in the
focuser, so we can still see the coma and work to remove it. Using
the coma corrector makes it much
easier to see the residual coma that we need to get rid of in
the "fine collimation" process.
Keeping the star centered,
defocus the star slightly
inside then outside of focus. Do it slowly at first, then
rapidly. Star testing like this is most sensitive when you are
very close to focus, so don't defocus by a large amount. You
should just start to see the secondary shadow emerge at most. If
you see it distinctly, you have probably defocused too much. This
will require only a fraction of a turn of the fine-focus knob.
Pay attention to how the star
expands, and be critical. Looks for small variations.
Let's
say that the star appears to "bulge" on one side on the inside of
focus, and
the "bulge" is still on the same side as you look at the image slightly
outside
of focus. This is likey a small amount of coma that needs to be
addressed by tilting the primary mirror a small amount.
(Pinched optics, or other distortions of the mirrors will
usually
"flip"
on the other side of focus, thus distinguishing them from coma.)
If
coma is not obvious, then the collimation is fairly good. In this
case, we need to get more critical. Don't just look at the shape
of the star after
you defocus it - observe how the star expands. That is, as
you
just barely start to defocus the star, watch to see if the star image
expands first in a particular direction, or "bulges" in that direction.
In doing this, we're looking to
see HOW the star expands, not
what it looks like when it's expanded. It is subtle at
first,
but
more obvious with practice. Doing this very close to focus
increases the sensitivity of the test.
To help with this, note
the location of the star at best focus, and stare at that spot.
Now defocus inside of focus and see if the star simply
expands in
all directions at once, or if it "bulges" or "pulls" in one direction,
expanding off-center.
Repeat going outside of focus. If you see the bulge
in the
same direction as going inside of focus, repeat by defocusing in both
directions a few more times to confirm that it is consistent and is not
a strange air current or moment of bad seeing. If it is
consistent, chances are it is coma.
Practice looking for small amounts of coma. You will get better
with time and practice, and small amounts of coma will become obvious
to you.
Now, if you see coma, either a lot of it or a little, use the primary's
collimation adjustments to move
the star toward the tail of the coma, which is in the direction of the
bulge that you see when defocusing.
This may be easier if you have a friend helping you by turning the
collimation bolts as you direct him/her to. Using the
primary's collimation adjustments to move the star tilts the
mirror so that the optical
axis (the spot
where a star will appear round even with no coma corrector being used)
gets closer to the center of the field of view of the eyepiece.
By making successive adjustments, you can put the
coma-free spot in the center of the eyepiece's field, and then the
telescope is nearly perfectly collimated.
NOTE: If you are working alone,
I
recommend first turning each bolt in both directions and making a "map"
of
how each one moves the star in the eyepiece. Then you can be
sure
of turning the right bolts to move the star where you need it, saving
time and frustration.
ANOTHER NOTE: If you can't distinguish the direction of the
coma,
or if the distortion does not seem to resemble the description of coma,
you may have more than one optical issue. The other issues
may
need to be fixed before you can more accurately collimate your
telescope.
Keep making adjustments until the star expands uniformly in
all directions, and does
not "bulge" or "pull" in one direction. At
this point your telescope will be fairly well collimated in terms of
the
primary's tilt, and you're ready to observe.
Check
collimation by this method throughout the night to see if it drifts,
and tweak if necessary. It is important to note that the
collimation of the telescope may shift
subtly or obviously as the scope is used throughout the night and/or as
it is moved up and down in altitude. These adjustments may be
required more than once each night to ensure optimal telescope
performance. They do pay off, particularly for planetary
images.
If you master this collimation technique, please tell your friends
where you saw it, and teach it to someone else.
A
little history - I learned star collimation out of
necessity.
Before I had really good lasers, etc. as alignment tools, it was the
only satisfactory way for me to nail the collimation of the fast
telescopes that I was using and show what the telescopes could really
do. (It is also the final step in collimating a classical
Cassegrain, of which I have built a few.)
I mainly used lasers because I found myself adjusting collimation after
dark, making an autcollimator difficult to use. So, over time I
moved away from the autocollimator and toward newer, higher quality
lasers that were accurately machined and held their own collimation
accurately over time.
Lasers have improved greatly (thank you Howie Glatter and others), but
still there are errors introduced by imperfect tools, the fit of the
tool in the focuser, and other small errors. No tool is perfect,
but some are better than others. So, the only method that avoids
all of the errors is final collimation using a star.
The method is self-regulating - on a night of poor seeing, if you can't
see the collimation error in the moving star image, it doesn't really
matter - the atmosphere is not going to reveal that level of detail
anyway. On a night of superb seeing, however, you are far more
likely to see errors in a star test after tools/lasers are used, and
that is when knowing how to tweak the collimation by the last little
bit using a star will allow those small errors to be removed, and the
utmost in performance can be reached. That is when the magic
truly
happens.
I can't count the number of times at the WSP when I would be up on the
ladder at Joe's 32" f/3.6 and I would see some collimation error.
This is due to changing temperatures and the imperfect structure of the
telescope. I would tell him what knob to turn, and images would
improve. Over time he just learned which way to turn the vertical
adjustment knob, and began to work on finding the sources of
error. While a barlowed laser gets the 32" collimated reasonably
well, it is still normal to have to tweak by using a star image.
Pinched optics
Pinched
optics means that the mirror is being squeezed by something.
Generally, since three points can locate a mirror laterally,
the effect of the "tightest" three screws are what is
seen, and that means a three-sided shape is created by the mechanical
distortion, which of course is a triangle. However, by
squeezing the mirror on two opposite sides, I have seen highly
astigmatic images created by pinched optics.
Pinched
optics have to be pretty bad to make a star look like a triangle, but
it happens!
The figure at right shows how star images may appear if one
of the optical
elements is pinched. More than likely you will only see a
hint of
triangularity
over a
basically circular shape. Chances are that it is being cause
by
an optic that was pinched during
manufacturing or that is being pinched in the telescope.
While it
may seem minor, you will want to track it down because it may become
worse at various temperatures, since metal and glass expand at
different rates, and the glass may be more severely pinched at
temperature extremes.
First,
inspect the mounting of the primary mirror. All clips and
other
retaining screws should not contact the mirror, but they should come
close. If you find a screw tightened against the mirror, even
a
small amount, back it off and re-test. You should be able to
"rattle" the primary in the cell. It should not be "snug" in
the cell, it should be slightly loose in all directions.
If no optics appear to be
pinched, then rotate the primary. If the "points" of the
triangle
rotate with the mirror, chance are the mirror was pinched during
manufacturing. In this case, let the mirror cool after it is
rotated and observe the star shape an hour or two later. If
the
points of the triangle go back to the original orientation, then the
triangularity is likely being cause by cooling, such as by three fans
or three ventilation holes.
If
the distortion does not rotate with the mirror, then it is possible
that the primary mirror's cell is distorting it. If the issue
is
poor edge support, then the problem will likely disappear if you point
the telescope straight up and ensure that the primary is not "stuck"
against the edge supports. Giving the scope a quick shake
(thereby "rattling" the mirror in its cell)
will
often cure that. Moving the scope back down near the horizon
would then cause the problem to return.
Often
mirror cells have only three edge supports, and if the mirror is heavy
enough, it can effectively become wedged between the two bottom
supports when the telescope is pointed low, and this squeezes the
mirror. (If the mirror is 10" or larger, I don't recommend a
three-edge-support cell.) This will cause triangular star
images and/or astigmatism as the telescope is pointed low in altitude,
and it may improve if the scope is pointed high. The mirror
may also get "stuck" a little bit and may remain pinched until it is
"rattled" in its cell.
Some mirror cells may actually pinch the mirror from time to time, due
to friction/stiction. That is, if the mirror sits on the cell
for
a long time, some of the contact points (plastic, fellt, cork) may
actually adhere to the mirror. The solution is simply to move
the
mirror in the cell, or rotate it slightly, just enough to break the
stiction, and get it "un-stuck". This may result in a
significant
improvement in the star test, and I have seen it cure a major problem
in a couple of cases.
Finally, check to see that the primary mirror is resting on all of its
rear support points and triangles. Occasionally part of a
cell
may bind up, or a part of a bolt or pin may push one or more triangles
higher than the rest, preventing the cell from hitting the back of the
mirror at all support points. Touch the back of the corner of
each triangle and see if it moves. The mirror should exert
equal
pressure on each of the cell's back support points, so if you find a
triangle or part of a triangle that is not touching the primary, that
is a major problem, even if it's only missing touching by a tiny
amount. Problems like this are one reason why people test
telescopes before delivering them to customers. They are
difficult to see without something approximating a mirror (a disk with
a very flat back) actually sitting in the cell, verifiying that all
clearances are correct.
It is possible
to have the secondary cause triangular distortion, but it is not
common. As a last resort, examine its mounting and see if it
is
being pinched. Like all optics, it should be held gently, and
not glued unless done by an experienced person.
What is
astigmatism?
Astigmatism
is an optical aberration. Mirrors with astigmatism produce
oval-shaped, or in terrible cases, line-shaped stars! The
oval or
line rotates 90 degrees as the eyepiece is moved to the other side of
focus. In mild cases, stars still generally appear round at
focus, though diffraction rings, if they are visible, may appear
distorted. In severe cases, the star will appear cross-shaped
at
focus. The figure above right shows what astigmatism may look
like in a telescope.
Primary
mirror astigmatism - polished in, and thermal
If
you notice astigmatic star images, the first step is to note the
orientation of the "ovals" on the inside and outside of focus.
Make sure that you record this with respect to the
telescope's
optical axis, and not the ground. That is, note the
orientation
of the ovals and which side of focus you are on with respect to the
tube of the telescope, not the ground. I tilt my head so that
it
is aligned with the tube - that is, an imaginary line drawn between my
eyes will be parallel with the telescope tube.
Now,
rotate the primary mirror. If the astigmatism rotates with
the
mirror, then the primary mirror is causing the astigmatism. HOWEVER, you must now
observe the astigmatism over several hours to see if it changes or goes
back to its original orientation.
If the astigmatism goes back to its previous orientation over
time, then chance are that you are seeing THERMAL astigmatism, likely
caused
by unevenly cooling the mirror.
Thermal astigmatism may appear or
disappear only on windy or still nights, so more than one evening of
testing may be required. If you see a pattern, look at how
the
cooling fans are positioned or the air flows through the mirror box due
to wind. Having only the bottom of the mirror box open at the
back may cool the bottom half of the mirror more, causing uneven
temperature. Having fans in a line rather than distributed
around
the mirror is also a usual suspect. The figure below shows
how I poorly
located the fans in a 16" f/4 telescope that I built some time ago.
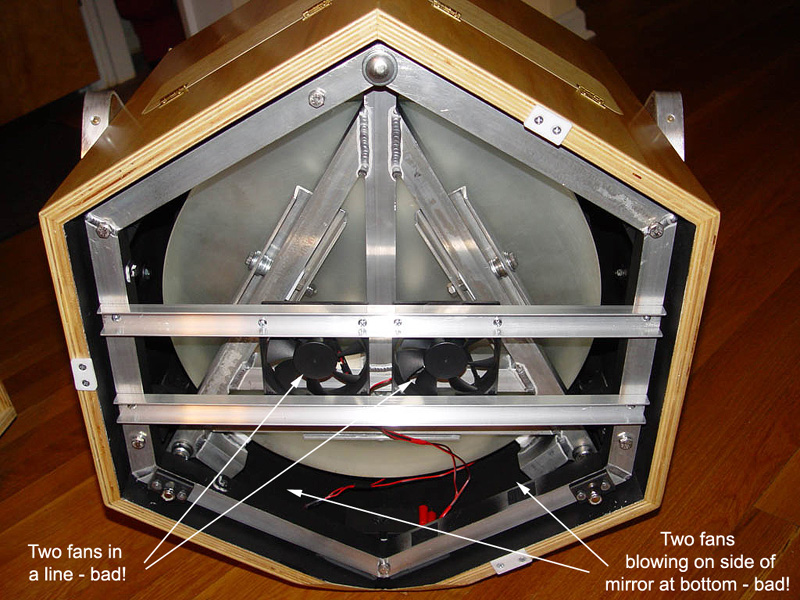
In all cases, though,
thermal astigmatism will appear to rotate with the primary at first,
but then it will eventually change or go back to its original
orientation.
If the astigmatism always follows the rotation of the primary
on
all nights and over many hours, it is being caused by the primary
mirror itself.
One form of thermal distortion that does not rotate with the primary
mirror is the effect of an air current. It will typically
distort
one side of a star image, but the effects can range from minor to
severe depending on the temperature differences involved. It
should be recognizable in out-of-focus star images as a slowly moving,
distorted area on one side of the image. In some cases, it
may be
much worse. A closed tube will make it worse, and pulling up
the
shroud on a truss-tube dob may help alleviate it and aid in primary
mirror cooling. It may also become less apparent as the
mirror cools over time.
It is also possible for a secondary mirror to show thermal astigmatism
while it cools. This is because the back of the secondary
mirror,
and the sides somewhat, are usually insulated by the holder, while the
optical surface can cool. This astigmatism will go away as
the
secondary mirror reaches equilibrium. Lastly, an over-active
dew
heater on the back of a secondary can also cause astigmatism.
Try
turning off the heater and see if the problem goes away.
Mirror-cell
induced astigmatism; Overheated, pinched and bad secondary mirrors
Let's
assume now
that you have checked, and the astigmatism does not rotate with the
primary mirror.
If
the ovals (defocused star images) appear roughly aligned with the tube
of the telescope, that is the oval on one side of focus is aligned with
the tube and on the other side of focus it is perpendicular to the
tube, then the likely culprits are a bad secondary mirror or the edge
support of the primary mirror. Tilting your head parallel or
perpendicular with the tube while looking at a star image can help you
determine if the astigmatism is aligned with the tube.
It's pretty obvious, but we call this alignment with the optical
axis because the optical axis is parallel to the telescope
tube. We also call this alignment
with gravity
because the force of gravity can cause astigmatism that is aligned with
the optical axis/telescope tube.
A mirror cell that is
causing astigmatism usually has inadequate edge support, such as a bad
sling or poorly selected contact points. It will pinch the
mirror
with the sling or push at incorrect places on the edge, squeezing or
bending the mirror. The astigmatism should change with
telescope
altitude, and if you point the telescope straight up and give it a
"good shake" to make sure the mirror isn't hung up on anything, then
the astigmatism should all but disappear.
A properly-positioned cable
sling should introduce a small amount of astigmatism in a primary
mirror, but not a large amount. If this is not the case, you
should make sure the cable is positioned properly at the center of
gravity of the mirror, and that the mirror
is not bumping into something else due to a slack cable.
If you
have astigmatism that actually seems to get better as the telescope is
pointed lower, then you may have a mirror cell with triangles or
supports that are bound up. I have seen this happen more than
once. As I mentioned in the Pinched Optics section above,
make sure the mirror is sitting on all of the support
points, and that the triangles or pivots can move freely. Either
of those will cause major problems with images.
If, after adjusting the cell, rotating the primary, shaking the scope,
or even giving the mirror box a good whack with your first, images
suddently become good and stars round, you have freed something that
was bound up. Pat yourself on the back, gloat to your
friends,
and have a beverage if you are so inclined. However, don't
forget
how you fixed the problem, and keep looking at the components of the
scope carefully. Eventually you'll figure out what is
actually
causing the problem.
Don't be surprised if the fix is as easy as bumping some part of the
scope to free it up. The moral of the story is this - you
don't
make stars round accidentally - you make them round by fixing problems.
A pinched secondary mirror can create oddly-shaped non-round
stars. This can happen when the shell of the holder pushes on the
edge of the secondary because the shell is too small or has the wrong
shape, or when there is too much force pushing the secondary into the
retaining lip, usually applied by the stuffing behind the secondary
mirror. In either case, the situation needs to be remedied.
For more information on recommend construction techniques for primary
mirror cells and recommended fitting techniques for secondary holders,
refer to my other article - A Modern Guide to Mirror Support.
A bad secondary
mirror will often have
a curve to its surface, and this can cause astigmatism because it is
mounted at a 45-degree angle. The astigmatism should be
fairly
consistent for all telescope altitudes, and will usually be aligned
with the tube. If
you can't find any other problems, have the secondary tested, or swap
it out and see if the problem goes away.
Additionally, a secondary mirror can be thermally warped into a curved
shape by a dew heater that is producing a lot of heat. That heat
is applied to the back of the secondary mirror, which cause it to
expand, making the shape of the front of the mirror concave, which
causes astigmatism. I have personally seen this occur on a large
6" m.a. flat in a 30" telescope. The heat plume coming off of the
mirror was conspicuous in the defocused image of a star, and that is
how I noticed the problem. After reducing the heat output we
watched the astigmatism disappear over about an hour. So, this
problem will not go away immediately after turning down the heat, but
smaller mirrors should respond more quickly.
Primary mirror strain-induced
astigmatism
If
the primary mirror is made from poorly annealed glass, it will have
strain. Strain is internal stress within the glass, and it is
more likely to be found in cheap mirrors made from cheap glass.
When
exposed to a temperature change, especially a rapid one such as
bringing a scope outside into the cold, the mirror may go astigmatic.
The
good news is, as the mirror temperature equalizes, the astigmatism
should go away. Additionally, the strain should rotate with
the
primary mirror and stay with its rotation over time as it slowly
disappears.
However,
if you like to observe shortly after bringing the telescope out, this
is not good news. You will be stuck with astigmatic images
until
the mirror cools sufficiently.
Poorly
aligned focuser or corrector
The
last cause of astigmatism that I have encountered is a poorly aligned
focuser or corrector. This can be a real problem for a faster
telescopes
(those with lower f/#s) and when coma correctors are used.
If all
other possible sources of astigmatism have been eliminated, check the
squareness of the focuser and make sure that the eyepiece and coma
corrector are in the focuser squarely. One time, I
experienced
a
case where the setscrew of a focuser pushed the coma corrector of to
one side and caused it to tilt slightly, introducing astigmatism and
other aberrations into the images of an f/4 telescope. I
fixed the problem by making sure the corrector was pushed all the way
into the focuser. As with a laser, make sure the top surface of
the focuser and the bottom surface of the corrector are clean so that
they can mate together cleanly, snugly, and precisely. Those
surfaces control the optical alignment of anything that is in the
focuser.
Naturally, after making sure that the coma corrector (hopefully a
Paracorr 2 for the faster, modern telescopes!) is properly inserted in
the focuser, one should also make
sure that all setscrews are tightened snugly. This includes the
two thumbscrews at the top of the focuser, the two thumbscrews at the
top of the Paracorr that hold the eyepiece/adapter in, and the
thumbscrew that locks the tunable top of the Paracorr. In total,
this is FIVE thumbscrews, and they are all important. They are of
even more importance when heavy and/or tall eyepieces are used, because
those eyepieces exert more torque on the Paracorr and focuser, and can
more easily become misaligned to the optical axis.
Side note: If you use the Starlight Instruments SIPS, which
includes a Paracorr 2 and eliminates the need to tune the Paracorr top,
then all you have to do is put the eyepiece in a focuser and tighten
its thumbscrews. This unit eliminates the possibility of having
the corrector in the focuser slightly askew, and, in my opinion,
contributes to the best possible image quality.
As a last resort, try
rotating the corrector and/or eyepiece in the focuser (making sure that
it is inserted all the way into the barrel) to see if the
astigmatism rotates with either of those. If it does, you
have
found your culprit.
Other
thermal effects on optics
Now
let's conclude this article by looking at one issue that involves round
stars. Purely thermal effects on optics aren't always of a
nature
that
causes stars to be non-round - often they affect the perceived
correction of the optic. Many people star test
telescopes to
help them determine the quality of their optics. However,
people
often mistake the effect of dropping temperatures on the
mirror
for optical problems.
The lower-cost glasses such as Pyrex, Supremax, plate glass, etc., have
coefficients of thermal expansion that are significant given typical
range of air temperature over the course of a night in most locations
on earth. This means that the mirrors actually shrink or expand
as their temperature decreases or increases. Also, it is normal
that certain parts of a mirror cool faster than others, so certain
parts of the mirror will shrink more quickly than others, and this will
temporarily change the optical figure of the mirror until all parts of
the mirror come back to the same temperature as the air.
Unfortunately this will never happen on most nights, and the amount of
difference varies.
The higher-cost
glasses and
materials such as quartz, Zerodur, Cervit, ULE, etc., have relatively
small coefficients of thermal expansion. This means that mirrors
made
from these materials don't change size or shape very much over a night,
making them very desirable for telescope mirrors. Unfortunately
quartz
is significantly more expensive than the lower-cost glasses, and the
others are far more expensive than that. Thus, most people have
mirrors made from the lower-cost glasses, so these mirrors change their
optical figure as the air around them changes temperature.
Therefore,
it is completely normal for a well-corrected,
high-quality mirror, made from Pyrex, Supremax, or other lower-cost
glass, to
appear overcorrected while it cools. This
condition is
indicated
by obvious rings in the structure of a defocused star on the outside of
focus, and indistinct rings on the inside of focus. As the
mirror
approaches air temperature, this condition gradually goes away.
The reason for this, as mentioned above, is the mirror doesn't
cool evenly -
it cools faster near the edge of the mirror and wherever the miror is
exposed to the cold sky above (obviously the front of the mirror is
exposed to the sky) or to cooling breezes or fans. The cooler
areas physically shrink, changing the shape of the mirror, and usually
resulting in perceived overcorrection as seen in the star test.
Thus, especially for larger mirrors star testing is climate dependent
since larger pieces of glass (generally 16" and up) cannot keep up with
the cooling of the air.
I have seen proof of this with my own eyes for years - for the two 32" telescopes
that we take to Okie-Tex and the Winter Star Party in the Florida Keys,
the star test at WSP is superb after a couple of hours. There
night time air temperatures don't fall much. However, the same
scopes star test overcorrected all night and sometimes shows a turned
edge (which is not there) in the desert environment of Okie-Tex.
This is true for both 32"s, so it is not a fluke.
So, not only are we testing the optics, we are also testing how the
glass changes shape as it cools. I figured the 32" mirrors to be very,
very close to parabolic, they are not purposely undercorrected.
So, I know that if I see a good star test for a large telescope in a
location where the air temperatures are falling quickly, then that
mirror is very likely undercorrected by a significant amount, or it is
made from a more expensive material (quartz, Zerodur, etc).
For a numerical example, let's say the outer part of a 2"-thick mirror
is one
degree Celcius (1.8 deg F) cooler than the rest of the mirror.
The coefficient of thermal expansion of Pyrex/Supremax
(borosilicate glass) is 3.25*10^-6 per degree C. If we assume
that the front of the mirror shrinks downward toward the center of the
mirror and the back of the mirror shrinks upward toward the center of
the mirror, then this means that we're interested in the dimensional
change of a 1" thick piece of glass (the 1"-thick layer of the primary
that lies between its center and the optical surface).
So, a one degree
lower temperature in the outer part of the mirror
means that the 1" of glass underneath the optical surface shrinks
downward by 3.25 millionths of an inch, or about 1/7-wave on the glass,
2/7-wave on the wavefront. This pulls down the outer part of
the
mirror, effectively overcorrecting it. The effect is visible
in a
star test and looks like overcorrection. It will affect
images a
bit.
A temperature difference of two degrees C, or 3.6 degrees F, results in
shrinking
by 2/7 wave on the glass, or 4/7 wave on the wavefront. This
should be very easily visible in the star test, and the effect on
images will be significant too. I have measured this much
distortion
in a 28" mirror that was tested in my shop while it was cooling
strongly, so I know that it is possible. (See my article Four Lessons in Mirror Testing to see those
measurements.)
To help you understand what is going on, I made the image below.
It shows
how the mirror changes shape due to internal temperature imbalance, the
type that is typically produced by cooling outdoor temperatures.
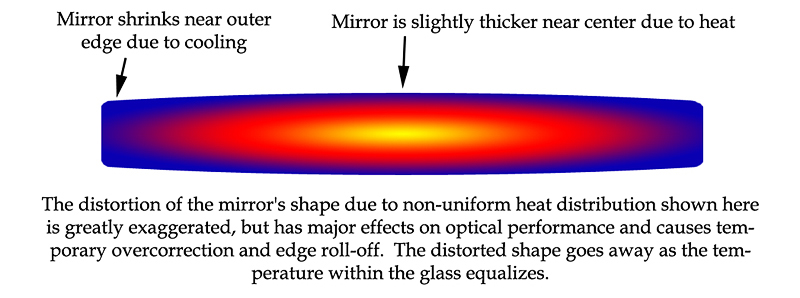
For many decades
it has been a common practice by some to leave mirrors made from Pyrex, Supremax, and higher expansion glasses
undercorrected to help
compensate for this, but that results in an inaccurate mirror when the
mirror temperature
gets close to equilibrium. These mirrors are then
undercorrected except when very specific observing conditions are experienced, and
will only perform optimally when cooling conditions exactly match the
amount of undercorrection that is figured in, which will be quite a
rare occurrence, and at that time the cooling itself will degrade the
performance anyway because convective heating will cause air currents. Doing this is quite unwise in my experience.
The implication of the information above is that star testing a large
mirror is not simple, and often it gives you an erroneous impression of
the quality of the mirror because accurately corrected mirrors appear
overcorrected while cooling. Therefore, star testing must be done
over many nights and in a variety of conditions in order to even get
close to estimating the true shape of the mirror. Even then, it
is my experience that a test done in a lab environment with everything
in thermal equilibrium is by far the best way to evaluate a telescope
mirror.
It
has been suggested by others in the past that insulating the edge of
the mirror could reduce temperature imbalance within the
glass, and may
help reduce the temperature-induced overcorrection. With the
lower-quality "light bucket" optics of the past, the effect of
insulating the edge may not have been noticeable or significant.
However, now that we are in
a modern era that features more high-quality, large, fast mirrors, it
may be time to try this again. I have not heard of anyone
trying it
recently, and I have not tried it myself since my 20" f/3.0 mirror is
only 1.25" thick, and the thermal effects are not as significant.
This brings the subject of mirror thickness into the discussion.
Thinner mirrors can be more difficult to make, and can require more
time and labor, thus making them more expensive. However, this
leads to faster cooling and better performance all through the night,
and also makes the telescope lighter. My recommendation for the fastest-cooling
mirror (the thinnest mirror, of course) that doesn't add significantly
to the cost of the mirror. For this, the ratio of mirror diameter to thickness is
approximately 12:1 to 15:1.
I often
use blanks that are 1.25" thick for 16" mirrors, 1.35" thick for 18"s,
1.4" thick for 20"s, 1.5" thick for 22"s, 1.6" thick for 24"s, and 2.0"
thick for 28"s, 30"s, and sometimes 32"s. However, this should
make it quite obvious that those who claim that 2.0" thick mirrors are
always better are simply saying that for marketing reasons, and it has
no basis in fact. The facts are that thinner mirrors, when made
properly and supported properly, will outperform thicker mirrors
because the thinner mirrors cool faster. This principle applies to any type of glass.
So,
thinner mirrors are better if you can support them properly, and quartz
isrecommended if it is available you can afford the extra expense.
Much higher-priced near-zero expansion materials like Zerodur,
ULE, etc., in my opinion are overkill for most amateur applications.
Getting back to thermal effects, metal
shrinks more than glass, so in some cases, a shrinking mirror cell can
actually pull very slightly on a mirror, particularly if the support
pads have even a slight tendency to "stick" to the glass.
This
may also
contribute to a
perceived overcorrection. Giving the mirror a "shake", as
described above, may
help in some
situations.
Finally, a client brought another thermal effect to my attention
recently. He noted that his secondary heater affected the star
test of a mirror that he had. This makes sense, because the
secondary heater may only heat the central part of the back of the
secondary mirror, and this can cause a variety of possible effects on
the wavefront. He noted that the secondary shadow "breakout"
position varied quite a bit on one side of focus versus the
other. This judging of the "breakout", or where the secondary
shadow becomes visible, is a popular way to help estimate the
correction of a primary mirror through star testing. However,
this observation can be made quite erroneous due to the thermal
condition of the primary OR secondary mirror, or BOTH.
Finally, one last note on correction issues that is not related to the
cooling of the mirror - check you Paracorr setting. I have seen
an improperly tuned Paracorr cause significant spherical aberration in
a star test on more than one occasion. This happens when the
eyepiece is changed and then tunable top is not tuned properly, or when
the proper 1.25" adapter is not used with certain eyepieces. For
reference, you can refer to the Paracorr Type 2 Instructions.
Bottom line - be careful with claims that you make based on star
testing. They might be quite wrong.
Conclusion
This
article has been many years in the making. I've experienced
all
kinds of telescope problems including most of the conditions that I've
described above. I've been filing
them away in my memory, and it was finally
time
to put them into an article. I've also
refined my collimation technique
that
I describe above so that I can always be assured of having good
collimation that lets very fast mirrors perform at their best, and
just as well as slower instruments.
So, the problems mentioned above can be very real, as are the
solutions.
As an optician, it is also in my
best interest to educate people on what can hurt or help the
performance of telescope optics. Quite honestly, educating
telescope owners reduces
the number of issues that I am blamed for, and allows their owners to
fix them and enjoy the full potential of their often very expensive
instruments.
I hope this article has helped you understand a bit more the behavior
of telescope optics under realistic conditions. I also hope
that
it has allowed you to improve your telescope's collimation,
and
has given you the skills to troubleshoot common problems with telescope
optics. If it has, please ask your friends to read it, and
teach
them what you have learned.
Next, I
recommend that you read my article "Four lessons in mirror testing"
for more information about a telescope that went through part of this
process, and more about related thermal and optical isues.
I wish you clear, dark skies, and round, tiny stars.
-Mike
Lockwood, Lockwood Custom Optics
|